Chlorella research report
- HOME
- Research report
- Chlorella research report
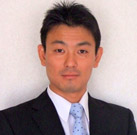
Faculty of Sport and Health Science, Ritsumeikan University
Motoyuki Iemitsu Professor
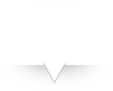
High-intensity intermittent exercise training with chlorella intake accelerates exercise performance and muscle glycolytic and oxidative capacity in rats
(Horii N, Hasegawa N, Fujie S, Uchida M, Miyamoto-Mikami E, Hashimoto T, Tabata I, Iemitsu M. High-intensity intermittent exercise training with chlorella intake accelerates exercise performance and muscle glycolytic and oxidative capacity in rats. Am J Physiol Regul Comp Physiol. 2017, Vo.312)
High-intensity intermittent exercise (HIIE) training (Tabata regimen)
The body has two energy delivery systems: "aerobic energy" that uses oxygen and "anaerobic energy" that does not use oxygen. Oxygen is used during exercise to produce energy from sugar and fat; however, in a short-distance running race where large kinetic energy is required in a short period of time, a metabolic mechanism called glycolysis works, which uses sugar as an energy source instead of oxygen. In the glycolytic system, lactic acid is produced as a metabolite, and as the exercise intensity gets closer to the maximum, lactic acid is increased and accumulated in the muscles. When lactic acid is accumulated more than a certain level in the muscles, the muscle acidity increases and people are no longer able to continue exercising.
The "aerobic energy" system can be trained with aerobic exercises such as jogging and aerobics, and as a result, endurance is increased. The "anaerobic energy" system can be trained with high-intensity interval exercises (for example in track and field, 200-meter runs 5 times).
Tabata regimen is a training program that can train two energy delivery systems simultaneously in a short period of time by giving 8 sets of intermittent exercise to exhaustion in a total of approximately 4 minutes, with 1 set of 20-second exercise and 10-second rest [References 1 and 2].
This study examined the effect of long-term intake of chlorella in combination with HIIE on muscle glycolytic and oxidative capacity as well as on exercise performance.
Methods
Male SD rats (12 weeks of age) were randomly divided into 4 groups (n=10): control (Con), chlorella intake (CH), HIIE training (HIIE), and combination of HIIE training and CH (HIIE+CH) group. The Con and HIIE groups received normal diet, and the CH and HIIE+CH groups received diet containing 0.5% chlorella powder for 6 weeks. During the 6-week study period, the HIIE and HIIE+CH groups underwent 14 sets of exercise 4 days a week: 1 set consists of 20-second swimming with bearing a weight of 16% of their body weight followed by 10-second rest.
Before and after the intake period, blood lactate levels were measured at rest (pre-HIIE) and 1 minute after HIIE (post-HIIE). The maximal number of sets of repetitions was measured using 1 set of 20 second swimming and 10 second resting, 48 hours after the last HIIE training of the 6-week study period. In addition, we determined the enzymatic activity of phosphofructokinase (PFK), lactate dehydrogenase (LDH), citrate synthase (CS), and cytochrome c oxidase (COX), and measured the expression of peroxisome proliferator-activated receptor γ coactivator-1α (PGC-1α), monocarboxylate transporter 1 (MCT1), and monocarboxylate transporter 4 (MCT4) in the red and white muscles of the gastrocnemius.
This study was conducted with the approval of the ethics committee for research involving animals at Ritsumeikan University.
【Results】
After the end of the 6-week intake period, blood lactate levels at 1 minute after HIIE were significantly lower in the HIIE and HIIE+CH groups than in the Con and CH groups, and significantly lower in the HIIE+CH group than in the HIIE group (Table 1). The maximal number of HIIE training was significantly higher in the CH, HIIE, and HIIE+CH groups than in the Con group, and significantly higher in the HIIE+CH group than in the CH and HIIE groups (Figure 1). The PFK activities in both red and white muscles were significantly higher in the CH, HIIE, and HIIE+CH groups than in the Con group, and significantly higher in the HIIE+CH group than in the CH and HIIE groups (Figure 2 A and B). The LDH activity was significantly higher in the CH, HIIE, and HIIE+CH groups than in the Con group, and significantly higher in the HIIE+CH group than in the CH and HIIE groups in the red muscle, but no significant difference was observed in the white muscle between the groups (Figure 2 C and D). The expression levels of PGC-1α in both red and white muscles were significantly higher in the CH, HIIE, and HIIE+CH groups than in the Con group, and significantly higher in the HIIE+CH group than in the CH and HIIE groups (Figure 3). The CS and COX activities in the red muscle were significantly higher in the CH, HIIE, and HIIE+CH groups than in the Con group, and significantly higher in the HIIE+CH group than in the CH and HIIE groups, but no significant difference was observed in the white muscle between the groups (Figure 4 A, B, C, and D). For MCT1 and MCT4, the expression levels in the red muscle were significantly higher in the CH, HIIE, and HIIE+CH groups than in the Con group, and significantly higher in the HIIE+CH group than in the CH and HIIE groups, but no significant difference was observed in the white muscle between the groups. The expression levels of MCT4 in both red and white muscles were significantly higher in the CH, HIIE, and HIIE+CH groups than in the Con group, and significantly higher in the HIIE+CH group than in the CH and HIIE groups (Figure 5 A, B, C, and D).
Table 1. Blood lactate levels pre- and post- HIIE training
Con (n=10) | CH (n=10) | HIIE (n=10) | HIIE+CH (n=10) | |
---|---|---|---|---|
Before intervention | ||||
Pre-HIIE | 1.97±0.18 | 2.03±0.31 | 2.18±0.34 | 1.92±0.39 |
Post-HIIE | 10.37±1.74 | 10.10±1.25 | 9.27±2.13 | 10.46±2.96 |
After intervention | ||||
Pre-HIIE | 1.88±0.12 | 1.87±0.18 | 1.77±0.16 | 1.70±0.22 |
Post-HIIE | 10.63±1.67 | 8.85±1.19 | 6.97±2.67#$ | 4.81±0.75#$& |
Values are expressed as mean ± SD. #P < 0.05 vs Con group at post-HIIE after intervention. $P < 0.05 vs CH group at post-HIIE after intervention. &P < 0.05 vs HIIE group at post-HIIE after intervention.
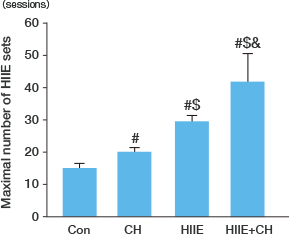
Figure 1. Effects of chlorella intake and/or HIIE training on maximal number of HIIE sets
Values are expressed as mean ± SD. #P < 0.05 vs Con group. $P < 0.05 vs CH group. &P < 0.05 vs HIIE group.
A
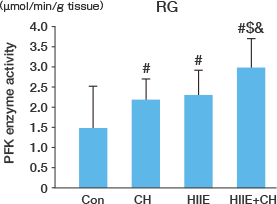
B
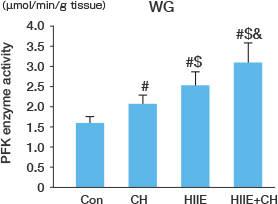
C
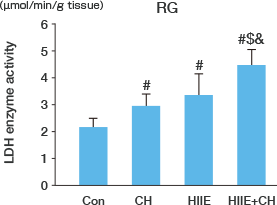
D
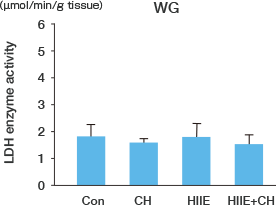
Figure 2. Effects of chlorella intake and/or HIIE training on PFK and LDH enzyme activities in the gastrocnemius muscles (red regions [RG] and white regions [WG])
Values are expressed as mean ± SD. #P < 0.0125 vs Con group. $P < 0.0125 vs CH group. &P < 0.0125 vs HIIE group.
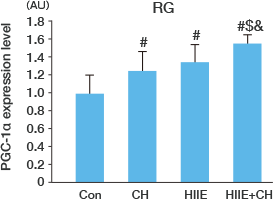
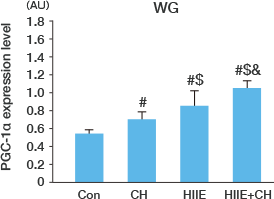
Figure 3. Effects of chlorella intake and/or HIIE training on the PGC-1α expression level in the gastrocnemius muscles (red regions [RG] and white regions [WG])
RValues are expressed as mean ± SD. #P < 0.0125 vs Con group. $P < 0.0125 vs CH group. &P < 0.0125 vs HIIE group.
A
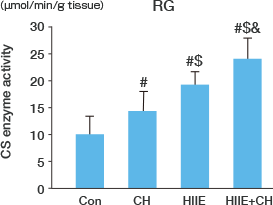
B
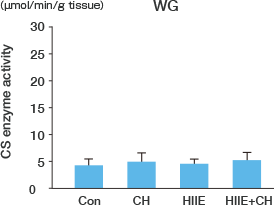
C
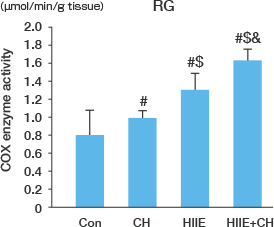
D
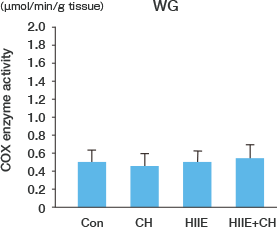
Figure 4. Effects of chlorella intake and/or HIIE training on CS and COX enzyme activities in the gastrocnemius muscles (red regions [RG] and white regions [WG])
Values are expressed as mean ± SD. #P < 0.0125 vs Con group. $P < 0.0125 vs CH group. &P < 0.0125 vs HIIE group.
A
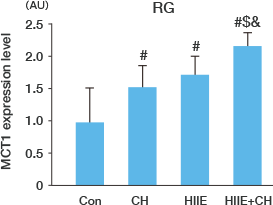
B
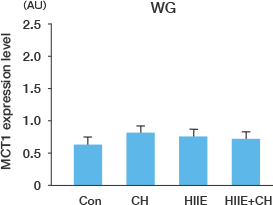
C
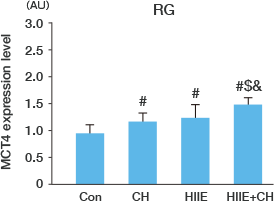
D
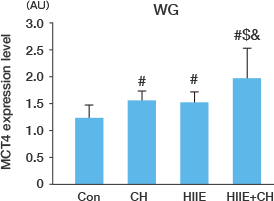
Figure 5. Effects of chlorella intake and/or HIIE training on MCT1 and MCT4 expression levels in the gastrocnemius muscles (red regions [RG] and white regions [WG])
Values are expressed as mean ± SD. #P < 0.0125 vs Con group. $P < 0.0125 vs CH group. &P < 0.0125 vs HIIE group.
【Discussion】
The combination of HIIE training and chlorella intake resulted in a synergistic increase in LDH, PFK, CS, and COX activities in the red muscle, and a synergistic increase in PFK activity in the white muscle. Furthermore, there was a synergistic increase in the expression of MCT1, MCT4, and PGC-1α in the red muscle and a synergistic increase in the expression of MCT4 and PGC-1α in the white muscle. These results suggest that the increased ATP supply capacity due to skeletal muscle glycolysis and oxidative metabolism improved aerobic and anaerobic exercise capacity.
The results of this study indicate that the combination of HIIE training and chlorella intake improves aerobic and anaerobic exercise capacity and promotes muscle glycolytic and oxidative metabolism, particularly lactate metabolism.
【Explanation of terms】
Monocarboxylate transporter 1 (MCT1)
It transports lactic acid. It is mainly distributed in the red skeletal muscle (slow muscle) and promotes uptake of lactate into cells, and then lactate is used for oxidation in mitochondria.
Monocarboxylate transporter 4 (MCT4)
It transports lactic acid. It is mainly distributed in the skeletal white muscle (fast muscle) and promotes lactate transportation from the cell to the extracellular space.
Phosphofructokinase (PFK)
It is a key enzyme in the glycolytic system that synthesizes acetyl CoA. The glycolytic system anaerobically synthesizes an energy substance (ATP). It is an indicator of the anaerobic energy supply capacity.
Lactate dehydrogenase (LDH)
It is an enzyme that synthesizes pyruvic acid from lactic acid in the final stage of the glycolytic system.
Peroxisome proliferator-activated receptor γ coactivator-1α (PGC-1α)
Its expression is increased in skeletal muscle by exercise, resulting in promotion of mitochondrial biogenesis.
Citrate synthase (CS)
It is one of the enzymes involved in mitochondrial energy production, and regulates the speed of the citric acid cycle, one of the aerobic respiratory metabolism pathway. A low activity of this enzyme reduces production of energy substances (ATP).
Cytochrome c oxidase (COX)
It is one of the enzymes that synthesize energy substances (ATP) in the electron transport system of aerobic respiratory metabolism. It is an indicator of the aerobic energy supply capacity.
Reference 1. Tabata I, Irisawa K, Kouzai M, et al. Metabolic profile of high-intensity intermittent exercise. Med Sci Sports Exerc. 1997, 29: 390-395.
Reference 2. Tabata I, Nishimura K, Kouzai M, et al. Effects of moderate-intensity endurance and high-intensity intermittent training on anaerobic capacity and VO2max. Med Sci Sports Exerc 1996, 28: 1327-1330.